The Earth is about 4.5 billion years old. If you apply a time analogy where the average human lifespan of 80 years is equivalent to 80 seconds, the age of Earth would be about 142.5 years. Although formed a long time ago, Earth is constantly changing, undergoing numerous reactions, transformations, and processes, each with vastly different time scales. For example, rock weathering processes can take millions of years, soil formation can take thousands to tens of thousands of years, while some microbiological processes in soil can take anywhere from a few days to several weeks.
The Earth has three main layers: the core, mantle, and crust. The core, composed of a solid inner layer and a molten outer layer made of iron and nickel, exists under extreme pressure and temperature. The mantle, above the core, is approximately 2900 kilometers thick and behaves both like a solid and plastic, with slow-moving currents. These currents drive the movement of the Earth’s thin crust, causing earthquakes and volcanic activity. The crust, home to all terrestrial life, is divided into oceanic and continental types. These crustal plates move slowly due to mantle currents, a process known as plate tectonics. Oceanic crust is denser and largely formed from volcanic materials, while continental crust consists of various rock types.
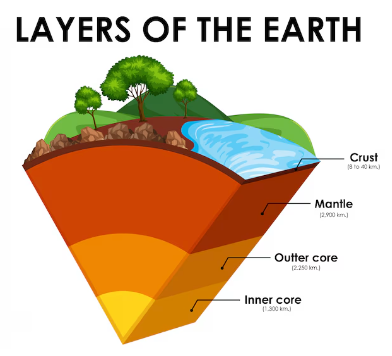
Minerals, the Earth's building blocks, are naturally occurring substances with specific chemical and physical properties, with nearly 5,000 identified minerals categorized into silicates and non-silicates. Silicates, such as quartz and feldspar, make up about 90% of the Earth's crust, while non-silicates, including halite, calcite, gypsum, and metals like gold and copper, though less abundant, are significant. Rocks form from specific combinations of these minerals depending on their origin.
Igneous rocks that solidify from magma deep within the Earth are known as plutonic or intrusive igneous rocks (such granite, gabbro), often resulting in large solid bodies called batholiths. In contrast, igneous rocks that solidify from molten rock extruding from a volcano are known as volcanic or extrusive igneous rocks (such as basalt, dacite), forming lava flows or ash fields that solidify into local or regional rock bodies.
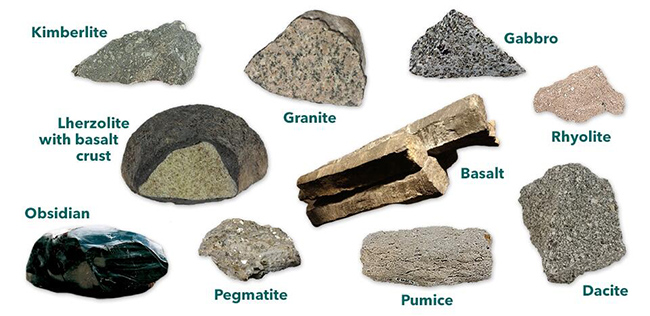
Clastic sedimentary rocks are formed from pieces of other rocks that have been eroded, transported by wind or water, and deposited in a new location. These sediments undergo lithification to become solid rock. Clastic rocks are classified by sediment size: gravel (greater than 2mm) forms conglomerates (rounded) or breccias (angular), sand (0.0625–2mm) forms sandstone, and mud (less than 0.0625mm) forms mudstone or shale. Most clastic sedimentary rocks are mineralogically homogeneous, with sandstone being predominantly composed of quartz. Biochemical sedimentary rocks form through biological processes. Common examples include limestone, composed of shell fragments and calcium-rich feces, coal, formed from plant material, and chert, formed from microscopic silica. Chemical sedimentary rocks form from mineral precipitation in water, often in environments like lakes or shallow seas with high evaporation rates. Examples include halite and gypsum, which form from evaporating saline water in places like the Great Salt Lake and the Dead Sea.
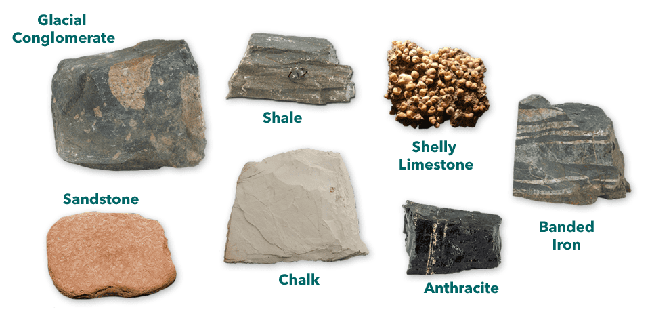
Metamorphic rocks are formed from existing rocks that undergo transformation due to heat and pressure, resulting in a complete metamorphosis into something new. They typically form deep under the Earth's surface where there is great pressure, often in regions with active plate tectonics. For example, many metamorphic rocks are found in the Appalachian region of the United States, where nearly 500 million years ago, the North American Plate collided with parts of the African Plate, creating a mountain system that once rivaled the Himalayas. Over millennia, immense pressure was exerted on the rocks deep beneath these mountains, and today, these metamorphic rocks are exposed in parts of the current Appalachian Mountains. Metamorphic rocks are commonly found in the great mountain ranges of the world. Sometimes, the link to the parent rock is clear, such as limestone metamorphosing into marble, sandstone into quartzite, and shale into slate. However, the origins of highly metamorphosed rocks like gneiss and schist can be more difficult to discern.
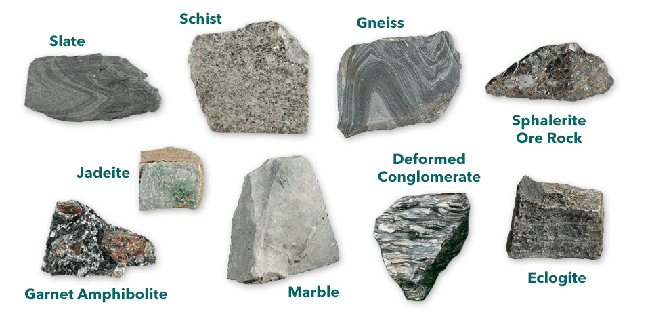
The rock cycle in Figure 2.9 (from the textbook) illustrates that the Earth is in a state of constant transformation due to natural surface and subsurface processes. Chemical and physical weathering, erosion, transportation, and deposition contribute to these changes. These processes are integral to the Earth's chemical and biological cycles, ensuring the recycling of Earth materials. In the current era, known as the Anthropocene, the rock cycle has been significantly impacted by rapid environmental changes caused by human activities such as the extraction of minerals, gas, and oil.
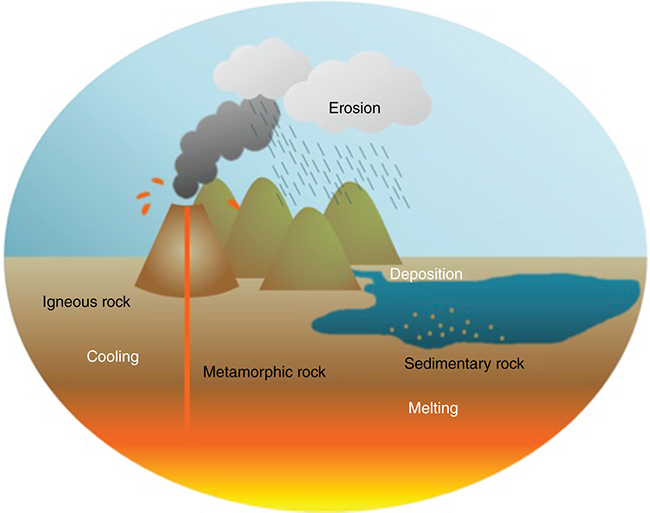
The extraction of natural deposits like minerals, gas, and oil is not considered renewable. These resources are finite and take millions of years to form, meaning they cannot be replenished on a human timescale. Once extracted and used, they are depleted and cannot be quickly replaced, unlike renewable resources such as solar or wind energy, which are naturally replenished over short periods. Unfortunately, renewable technologies require mining of a few non-renewable elements from the Earth.
When examining the elemental composition of the Earth’s crust (Figure 2.10), we find that only eight major elements account for approximately 98.8% of it, while all other elements on the periodic table together make up about 1% of accessible resources. As we see, elements used in high-tech applications are relatively scarce.

Click to expand to provide more information
Element | Approximate % by Weight |
---|---|
Oxygen | 46.6 |
Silicon | 27.7 |
Aluminum | 8.1 |
Iron | 5.0 |
Calcium | 3.6 |
Sodium | 2.8 |
Potassium | 2.6 |
Magnesium | 2.1 |
All others | 1.5 |
That simple fact has enormous implications for the availability of resources. Geological facts contradict the assumption that some natural resources are and will remain abundant. It also contradicts the assumption that man-made resources can readily substitute these scarce resources. This is due to an interesting fact about the periodic table: elements with similar properties that can substitute for each other in man-made devices tend to have similar chemical and geochemical properties. Thus, if one of these elements is scarce, elements with similar properties also tend to be scarce. While technological development has limited capacity to overcome resource scarcity, designing new products with resource scarcity in mind should be one of the goals of sustainable development.
In the next section, we will look into Earth's critical materials that are commonly used for renewable energy applications.