Obduction Zones: The Push-Together Boundaries
The Smokies are a small part of the great Appalachian Mountain chain, which extends along the coast of North America from Newfoundland through the Smokies, and then bends westward into Oklahoma. In the Great Smokies, the mountains display a truly remarkable feature—in some places, older rocks sit on top of younger rocks! The very high peaks are composed of hard, resistant, old metamorphic rocks (which we will explain soon), of the sort that one finds deep in a mountain range. Beneath them are younger, sedimentary rocks that were deposited in shallow seaways. Between these is a surface called a thrust fault or push-together fault. Thrust faults often show scratches that form when the rocks on one side of the fault slide past the rocks on the other side. Thrust faulting has been observed during earthquakes in some cases elsewhere in the world where push-together deformation is still active. In the Smokies, the older rocks have been shoved as much as 70 miles (110 km) to reach their present position on top of the younger rocks. The pictures below the video show two very much smaller thrust faults, with the upper rocks shoved up to the left only a few inches, but the idea is the same.
Video: Cades Cove (4:21)
Dr. Richard B. Alley: So this is a black bear walking down into Cades Cove in the Great Smoky Mountains National Park. The Smokies formed by obduction. They were formed in a great collision. And you know something about collisions. If this car worked before the Collision, it would have had a front that was fairly long horizontally and fairly short, thin vertically. But when it ran into the wall, you can see what happened. It got squeezed horizontally and thickened vertically. So it's shorter horizontally, thicker vertically. And this one did it by bending and getting squeezed and so on. In the Collision of the Great Smoky Mountains, there was some such bending. But, in addition, older rocks were shoved on top of younger ones, as shown in this diagram where the bear is walking, and we're gonna look at how that happened. First just a little geology terminology. Here's a pile of notebooks and things at our house. And to a geologist, you'd look at the one on the bottom and say that was put there first, it's been there longer, it's an older layer. And the one on the top was put there more recently, so that's a younger layer. Well now let's go to Glacier National Park, and this is a great cliff in Glacier, and we're going to zoom in on this cliff. And what we have here is a pile of layers, something like those notebooks. The one on the bottom is put down first, it's been there longer, it's an older layer. The one on the top was put there more recently, so it's a younger layer. But you'll also notice that if we follow this layer along, how do we find it? Well, we have to go up there, because there's been displacement here, the rocks were squeezed, and the squeeze broke the rocks, to make what we call a fault. And so you have this pile of rocks on both sides, but there's been some shoving going on. And we can put some labels on this to make it a little easy. On the left, you have the older at the bottom, and the younger on the top. On the right you have the older at the bottom and the younger at the top. But right here, notice what happens, the older is sitting on top of the younger. So now that allows us to go back and make sense of where the bear is. So here we are back at the bear, and he is walking through this diagram, with the older rocks on top of the younger. How did it get there? Well, let's do a simple diagram. Here it's older on the bottom, younger on the top, it gets squeezed by push-together stresses, and it's going to break along a fault like this. Okay, now faults sometimes move the rocks up a lot vertically and a little horizontally. The one at Glacier was pretty steep. The fault where the bear is moved mostly horizontally but with a little bit of vertical. And so it moved them a long way horizontal. So, I want you to focus on the "Y" in the younger layer and the "O" in the older layer, and when the fault breaks the O is going to go over by the Y, and you're going to pile it up like this. Well after that happened, after the fault broke, and it moved it like this, erosion happened, and it took down the surface, so it looked something like that, where the bear is. Or it looked like this diagram we've been looking at, or it looked like the bear. And so there we have push-together can bend things, it can squeeze them, but it can also shove older ones on top of younger ones so the bear can be walking on the younger rocks at Cades Cove in the Great Smokies.
You may recall that we started with pull-apart faults at Death Valley. As shown in the video above, thrust faults are of the push-together type. Squeezing from the sides caused one set of rocks to be pushed over another set. Each set is right-side up, but where they meet, the older rocks are on top of the younger ones. This is seen clearly in the Great Smokies.
Farther north, near Penn State’s University Park, Pennsylvania campus, where Drs. Alley and Anandakrishnan teach and where Dr. Alley wrote most of this material, we see a different way that rocks can respond to push-together stresses. There, in addition to some push-together thrust faults, many folds occur. Take a piece of paper, lay it on your desk, and squeeze the opposite sides towards the center. The paper will buckle into a fold. You may achieve the same effect by trying to push a carpet along the floor. Clearly, there are push-together forces involved here.
Watch this 1:38-minute video about the push-together thrust faults found in Capitol Reef National Park.
Video: Monocline Explained: Capitol Reef National Park (1:38)
[MUSIC PLAYING]
Dr. Richard B. Alley: We're at Capitol Reef National Park, home of the famous Waterpocket Fold. In the background, we can see it. This is a classic monocline, and it's a 100-mile-long flexure in the Earth's crust. And Dave's going to help show us how it formed.
This is the Earth's crust. And then 50 to 70 million years ago compressional stress came from the west and caused a bulge. And rocks on the west side were lifted up on along a thrust fault about 7,000 feet higher than rocks on the east side. Later, uplift during the uplift of the Colorado Plateau about 15 to 20 million years ago left it susceptible to erosion, and the top part was eroded off. And you got many of the geological features you'll find in the park, like monoliths, and canyons, and arches.
The reason the Waterpocket Fold got its name is when the younger layers up here were eroded, softer layers beneath were exposed, and formed small basins in which water gathered in, which provided a water source for early settlers.
[MUSIC PLAYING]
These photos show two small thrust faults, with one bed of sandstone thrust a few inches over another in each fault, and Dr. Alley’s index finger for scale, in a cliff below the Glen Canyon Dam in Arizona. The same principles apply to thrust faults, whether they are tiny or huge —push-together forces shove some rocks over other rocks along a break in the rocks. The rocks were squeezed from left and right, and the yellow arrows show the motion along the faults, which are indicated in white. The turquoise line segments were connected end-to-end as one straight, horizontal line before the rocks moved along the faults.
Virtual Field Trip: Blue Ridge Mountains
To see more on obduction zones, look at the Blue Ridge Mountains VTRIP. It has some pretty pictures, and then a little geologic background. There is another thrust fault, shown by yellow arrows, in the second-to-last picture.
We saw that pull-apart forces occur at spreading ridges. And, if rocks can be pulled apart, they can be pushed together, with push-together forces at subduction zones, or other collision zones. Today, you can look from the Appalachians and from the east coast of South America across the quiet seafloor of the Atlantic, across the spreading center of the mid-Atlantic ridge, to the coastlines of Africa and Europe. The coastlines on either side of the Atlantic are nearly parallel to each other and the mid-Atlantic ridge—slide the new and old worlds back together again, and they fit like a jigsaw puzzle. You can put all the modern continents back together jigsaw puzzle style. This fact, and especially the wonderful fit across the Atlantic, has figured prominently in suggesting the idea of drifting continents to scientists and other observers almost since the first decent maps were available of the Atlantic coasts. More importantly, putting the continent shapes back together in jigsaw-puzzle style puts the “picture”—the geology—back together, as well, for events that happened while the continents were joined. For example, we will see in Module 7 that, if glaciers flow over rocks and then melt, scratches are left that show which way the glacier was flowing. These tracks from a long-gone glacier run out into the Atlantic from Africa, and then out of the Atlantic onto South America. But if you put the continents back together jigsaw-puzzle style, the tracks fit together to show the path of a single glacier from a time when the Atlantic Ocean did not exist. Many other such matches are seen, from the times after the proto-Atlantic Ocean closed and before the modern Atlantic Ocean opened.
The oldest rocks on the Atlantic seafloor are about 150 million years old, approximately the same age as sediments that were deposited in a Death-Valley-type setting in the Newark Basin of New Jersey and elsewhere along the U.S. East Coast. The modern situation of a spreading Atlantic began about then, splitting apart a supercontinent to form the Atlantic Ocean in the same way that Baja California is being split off to open the Gulf of California.
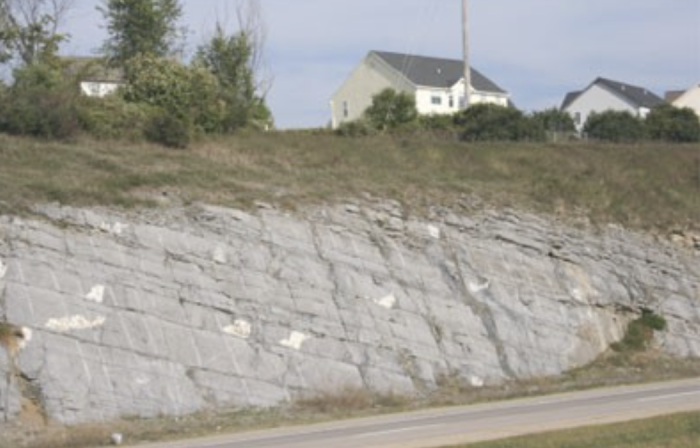
But the Appalachian Mountains are much older than that. A story begins to emerge of a cycle—older push-together forces led to the closing of a proto-Atlantic Ocean that produced the Appalachians. When the proto-Atlantic was closing, subduction-zone volcanoes formed and spread ash layers across the land, much as Crater Lake/Mt. Mazama and Mt. St. Helens did more recently. (Some of those ash layers can be found in many places, including the road cut along the Route 322 expressway just south of East College Avenue in the State College, PA area). Sometimes, the proto-Atlantic subduction zones formed offshore, formed volcanic island arcs, and then their volcanoes moved and collided with the North American continent.
When the pushing stopped, the giant pile of the Appalachians, with deep, hot rocks beneath, began to fall apart in Death Valley style, and red sandstones and mudstones accumulated in Death Valley-style valleys along parts of the U.S. East Coast. Some of these have interesting fossils, such as the dinosaur tracks at Dinosaur State Park in Connecticut; the real tracks are shown in the picture with an artist’s rendition of how the landscape might have appeared. The drop in pressure deep in the earth as the Appalachians fell apart probably caused a convection cell in the deep mantle to rise right there, eventually forming the mid-Atlantic Ridge and the Atlantic Ocean.
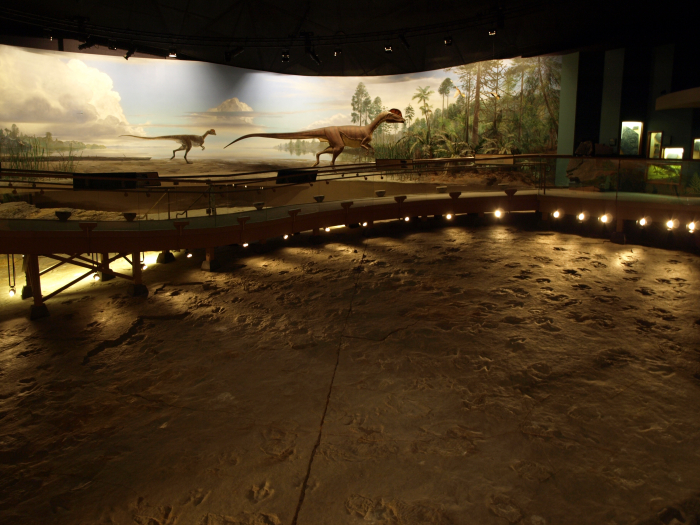
Such a cycle, with subduction or continental collisions building mountain ranges that then spread Death-Valley style and eventually split to make ocean basins, has been played out many times over the history of the Earth. And the fun isn’t over yet; North and South America are cruising westward toward Australia and Asia, as the Atlantic widens and the Pacific narrows. Africa is still bumping into Europe and pushing up the Alps, and India has not yet grown weary of ramming Asia to raise the Himalayas. At fingernail-growth speed, the next 100 million years or so should lead to a lot of geological high drama, but the next 100 years won’t see a whole lot of change. The 1:15 minute silent animation below provides a good visualization. (The animation would be more accurate if it made the crust a little thicker in the collision at the end, but otherwise, it is quite good. The animation shows that after the ocean spread for a while, the sea floor near the continent became cold enough and thus dense enough to start a new subduction zone. That might happen on one or both sides of the Atlantic in the future.
Video: Geology: Wilson Cycle (1:15) This video is not narrated.
On-screen text describes what is being shown in the video, as follows:
- continental rifting
- formation of spreading center
- opening of ocean basin
- onset of subduction
- continental collision
Additional on-screen text about the artist and animator:
- Wilson Cycle, animation by ARTEMIS@MIT, web.mit.edu/artemis
- Artist and animator, Krista Shapton
- Geology advisor, Taylor Perron
- Produced and directed by Violeta Ivanova
- Distributed under Creative Commons, Attribution-NonCommercial-NoDerivs, United States, 3.0 License.
The key to most of this is that you can sink old, cold sea floor, but you can’t sink a continent. Island arcs and continents float on the mantle too well. So rather than going down the subduction zone with the oceanic lithosphere, an island arc or continent will ride across the subduction zone for a major collision. In such a collision, called obduction, layers of rock are bent into folds such as those near Penn State’s University Park campus, or broken into thrust faults such as those under the Blue Ridge and the Great Smokies. In the case of the Appalachians, the thrust faulting was very efficient, with older rocks sliding tens or hundreds of miles (or kilometers) over younger ones in some places.
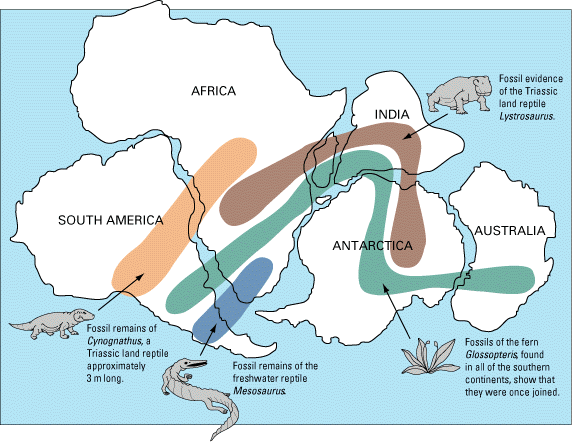
To see drifting continents in the past and the future, see the video 240 million years ago to 250 million years in the future.