Prioritize...
When you've completed this page, you should be able to describe the conditions that favor the development of mountain-valley circulations, as well as describe the nature of these circulations both during the daytime and at night. You should also be able to describe the up- and down-valley winds that can result from a secondary circulation, and discuss the forecasting impacts of these circulations.
Read...
When the prevailing synoptic-scale pressure gradient is weak, the differential heating and cooling of the earth's surface often promotes circulations that run counter to the large-scale wind flow. In mountainous regions this terrain induced circulation is called a mountain-valley circulation.
Daytime Mesoscale Circulations
The set-up for a daytime mountain-valley circulation hinges on differential heating. Incoming solar energy strikes east- and south-facing slopes sooner and more directly than the relatively flat, adjoining valley. In effect, this differential heating creates a local hot spot on the mountain slope. In other words, the air in contact with the mountain is warmer than air not in contact with the mountain at the same altitude. Now focus your attention on the sample air column shown in the top schematic (below) and keep the generic animation you just reviewed in mind. Note, near the bottom of the air column, how the 900-mb pressure surface dips toward the mountain slope, indicating lower pressure (also notice that the 900-mb pressure surface remains relatively flat over the valley). Meanwhile, higher up in the air column, developing higher pressure sets the stage for air to move away from the mountain. Over time, solar heating establishes a dry adiabatic lapse rate in a layer roughly one kilometer deep surrounding the mountain (the boundary layer) and air in the boundary layer becomes positively buoyant and starts to rise. The end result is that a thermally direct mesoscale circulation develops (bottom schematic shown below).
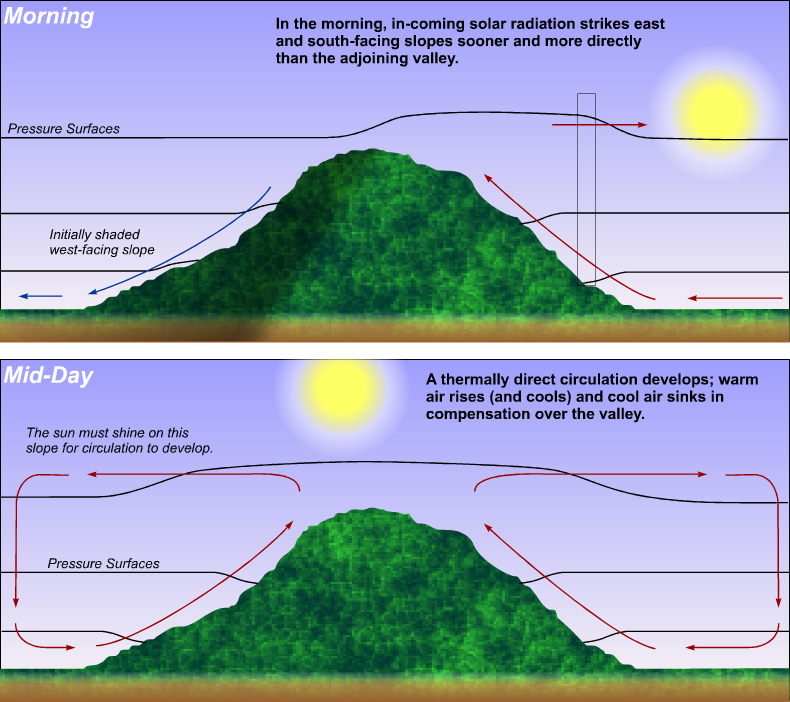
The compensating subsidence over the valley now explains the clearing we previously observed in the river valley south of the Black Sea. I also point out that the mountain-valley circulation along initially shaded west-facing slopes begins later in the day (shaded north-facing slopes are pretty much exempt from this discussion).
Now for the tale of the tape. As a general rule, the daytime upslope flow associated with the mountain-valley circulation is typically several hundred meters deep, and upslope speeds typically average about 3-6 meters per second (approximately 6-12 knots).
With our daytime mountain-valley mesoscale circulation established, our story isn't quite finished, however. Indeed, there's a secondary circulation that can develop, that results in something called an up-valley wind. The pattern of upslope winds associated with the mountain-valley circulation then creates an area of low-level divergence that, in turn, promotes an up-valley wind. By "up-valley," I'm implying that the valley floor isn't perfectly flat (which is true in most cases). I emphasize here that there are really two distinct circulations of air during the daytime (check out the schematic below). Note the up-valley wind at the surface and the counter flow of air aloft that help to complete a circulation of air whose axis parallels the valley axis. In other words, the circulation associated with the up-valley wind is superimposed on the mountain-valley circulation. Clearly, the two circulations go hand in hand, with their formation optimized when synoptic-scale pressure gradients are weak and the early daytime sky is generally clear, which favors differential heating.
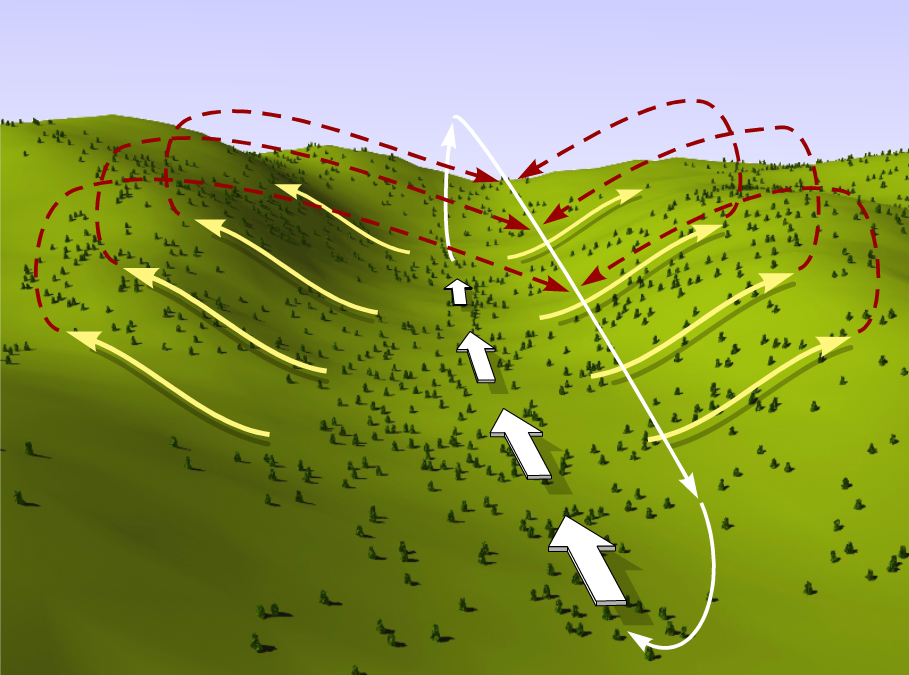
At night, the situation changes as the land surface along the mountain begins to cool. Let's investigate.
Nighttime Mesoscale Circulations
At night, net radiational cooling of the mountain slope causes the overlying air to cool by conduction. In turn, the dense air sinks down the mountain slopes, creating drainage winds (sometimes called katabatic winds). Check out the image below, which shows mountain drainage winds at night and the corresponding mountain-valley circulation of air (note that the nocturnal inversion over the valley governs the depth of the circulation). Not surprisingly, katabatic winds flourish when the synoptic-scale pressure gradient is weak and the nighttime sky is clear. In other words, these conditions set the stage for pronounced net radiational cooling of the mountain slopes.
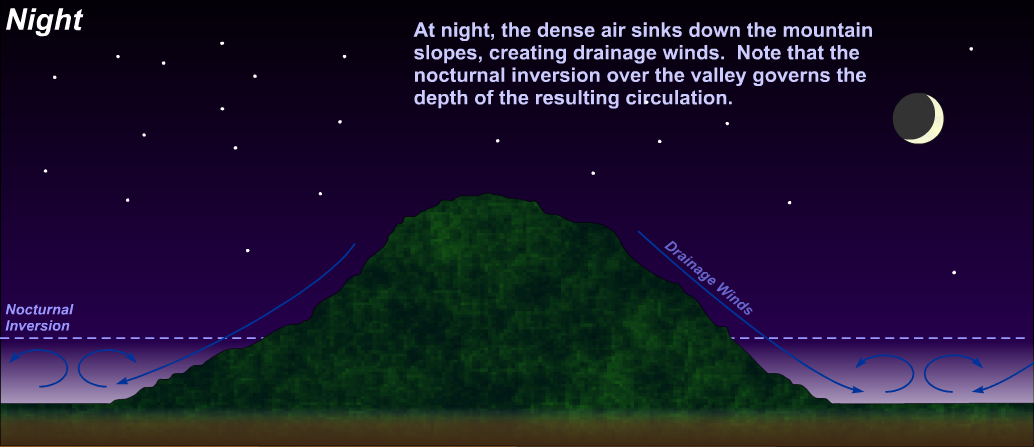
Of course, cold, dense air draining down mountain slopes does warm some by way of adiabatic compression during descent, but not enough to offset net radiational cooling. Because drainage winds move relatively slowly, the rate of adiabatic warming is usually less than the effects of net radiational cooling. For the record, the typical speed of drainage winds ranges from one to five meters per second (approximately 2-10 knots). I note, however, that the speed of nighttime katabatic winds can sometimes exceed 8 meters per second (16 knots), depending on the local terrain (steepness, etc.) and prevailing weather conditions. As you might expect, the down-valley wind tends to be stronger in deeper valleys.
In special cases, cold-air avalanches, which occur when cold air builds up on plateaus and then spills downward into the valleys below, can attain speeds of several tens of meters per second. That's really fast in comparison to typical cases! Remarkably, cold-air avalanches can occur more than once during a single night. To get a visual for how a cold-air avalanche can occur, check out this animation showing an idealized cold-air avalanche from the Cooperative Program for Operational Meteorology, Education, and Training (COMET) University Corporation of Atmospheric Research.
The convergence of drainage winds into the valley below paves the way for a secondary mesoscale circulation of air that results in nighttime down-valley winds. Like its daytime counterpart, the mesoscale circulation of air associated with the down-valley wind is superimposed on the mountain-valley circulation driven by the nocturnal cooling of the mountain slopes. For the record, the nocturnal low-level inversion over the valley governs the depth of the down-valley wind (the nocturnal inversion is typically as deep as the valley).
As you might suspect, the depth of the drainage flow of air is usually less than the depth of the upslope flow associated with the daytime mountain-valley circulation. Indeed, the layer of air cooled by conduction at night is not nearly as deep as the heated layer of air overlying the mountain slopes during the daytime. On average, the depth of the nighttime drainage flow is on the order of several tens of meters.
To see up- and down-valley winds in action, check out the animation (below) showing the evolution of the surface winds representative of a clear summer day in Utah's Tooele Valley. For the record, the prevailing synoptic-scale pressure gradient was weak and the overall synoptic-scale pattern did not change over the region during the course of this representative day.
The reason for the well-defined wind shifts from nighttime to daytime were up-valley and down-valley winds. The floor of the Tooele Valley is not flat, and during the night and early morning, southerly winds blew downhill toward the Great Salt Lake. During the afternoon, northerly winds blew uphill away from the Great Salt Lake.
In addition to changing wind directions, there are some other forecasting issues associated with up-valley and down-valley winds that you need to be aware of. When dealing with up-valley and down-valley winds, forecasters need to consider:
- Stronger daytime and nighttime winds than you might anticipate, given the prevailing weak synoptic-scale pressure gradient
- Higher nighttime temperatures than you might expect on a clear night and a prevailing weak synoptic-scale pressure gradient (remember that mechanical eddies mix warmer air to the ground)
- Light turbulence (a forecasting issue for small aircraft)
- Mountain-top convection during the daytime
Case Study...
When the prevailing synoptic-scale pressure gradient is weak, the differential heating and cooling of the earth's surface often promotes daytime up-valley winds and nighttime down-valley winds in mountainous regions. While you just saw an example of up-valley and down-valley winds during the summer near Salt Lake City, Utah, up-valley and down-valley winds occur throughout the year in mountainous regions. For a cold season example, let's look at Grand Junction, Colorado (KGJT), which lies in the Grand Valley in the western part of the state (here's a topographical map of Colorado for perspective). Please note that the Grand Valley (within which Grand Junction lies) has a northwest-to-southeast orientation. That's an important distinction to make because this local lay of the land has a dramatic impact on the prevailing wind direction at GJT, particularly when the synoptic-scale pressure gradient is relatively weak. To see the impact of the orientation of the Grand Valley on the prevailing wind direction at GJT, check out the wind rose for November. Note the maximum frequency of southeasterly winds and the secondary relative maximum in northwesterly winds. Clearly, the lay of the land impacts the distribution of local wind directions.
On the night of November 23, 2006, an area of high pressure governed the central Rockies and the eastern half of the central Great Basin (check out the corresponding 06Z analysis of mean sea-level pressure). Of course, elevations near Grand Junction are nowhere near sea-level. They're closer to 850 mb, so to get a sense for the gradient at 850 mb, here's the corresponding 850-mb analysis at 06Z. It's clear that the synoptic-scale pressure (height) gradient was relatively weak and that, at face value, the overnight winds should have been relatively light. Now turn your attention to the meteogram below, spanning 04Z on November 23 to 05Z on November 24 (below).
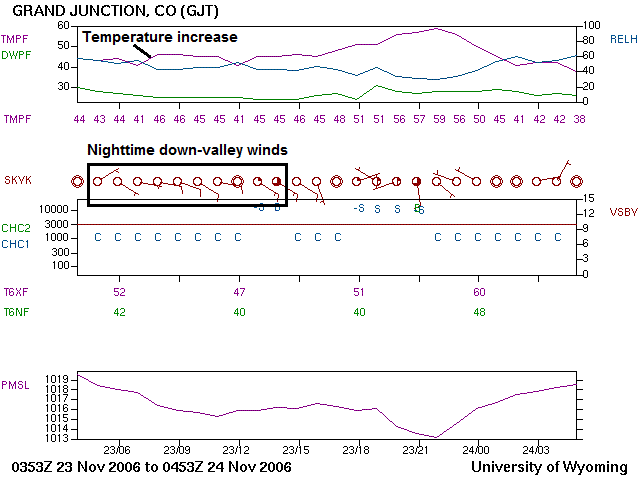
Note the calm wind at 04Z, which was consistent with the prevailing weak synoptic-scale pressure gradient. The down-valley wind at Grand Junction usually starts to blow from the northeast early in the evening, and then turns out of the east and then becomes southeasterly during the night. As you can see from the meteogram, that's what happened here, and by 08Z (1 AM local time), wind speeds were near 10 knots. Around 13Z (6 AM local time), the down-valley wind reached speeds near 15 knots. Not too shabby for a weak synoptic-scale pressure gradient, eh? Such is the power of the down-valley wind associated with the mesoscale circulation of air generated by differential cooling at night.
The sky was clear on this night, which favors differential cooling along the mountain slopes. Also note that the temperatures actually began to rise at GJT after 06Z as wind speeds increased, thanks to mixing from mechanical eddies brining warmer air to the surface. So, down-valley winds can be important factors in nighttime temperature forecasts.
Of course, as I mentioned before, daytime up-valley and nighttime down-valley winds can occur at any time during the year. For a warm-season example at Grand Junction, check out the evolution of surface winds on the meteogram for July 24, 2006. This was another day when a high-pressure system over the Great Basin provided a clear sky and a weak synoptic-scale pressure gradient. The meteogram for Grand Junction shows a clear transition from daytime up-valley winds (from the northwest) to nighttime down-valley winds (from the southeast) as day turned to night. Speeds associated with the nighttime down-valley winds approached 20 knots! Not surprisingly, mechanical eddies kept temperatures from dropping much overnight. I should also point out that in relatively deep valleys like the Grand Valley (where GJT is located), the reversal from down-valley winds to up-valley winds can occur as late as early afternoon.So, don't be lulled into the idea that there will necessarily be little wind in mountainous regions, even when pressure gradients are weak. Now that we've covered mountain-valley circulations and their impacts, let's explore the role of mountains in initiating deep, moist convection.